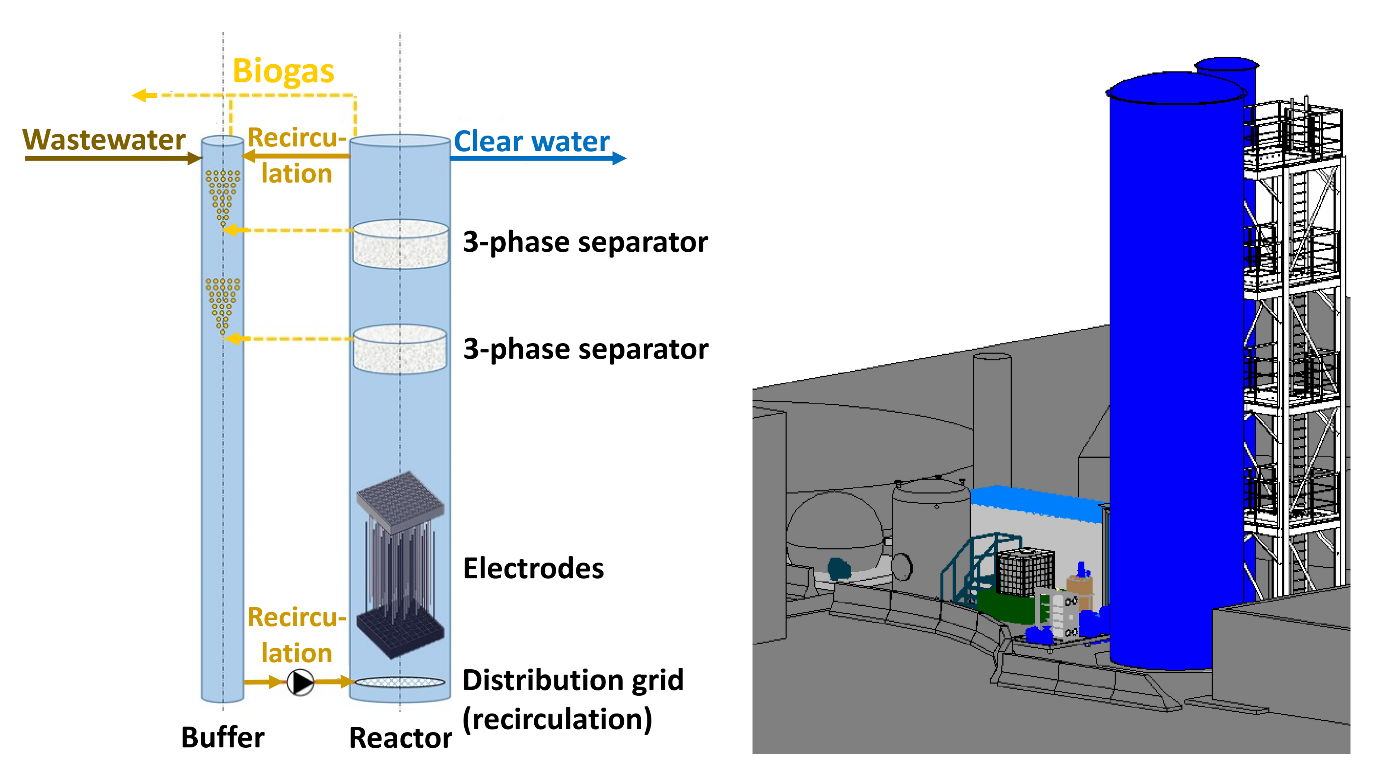
Unique selling points
- Energy recovery in the form of biogas
- Higher organic loading rates compared to anaerobic digestion
- Increased process stability
- Short stabilisation periods after shock loads
- Higher calorific value of the biogas due to higher H2 content
Description of the technology
The electrostimulated anaerobic reactor (ELSARTM) combines anaerobic digestion with bioelectrical processes. It can be applied as anaerobic pretreatment of raw wastewater with high COD loads or as sludge treatment. Until now the majority of bioelectrochemical processes have only been operated in lower scale configurations (Park et al. 2020a). In Ultimate, one of the first industrial scale system treating 450 m³/d of wastewater is demonstrated (CS Lleida). The reactor is basically a combination of an upflow anaerobic sludge blanket reactor (UASB) with integrated electrodes (Fig. 1).
Here, organic matter is converted to biogas by fermentative and methanogenic microorganisms. Due to the integrated bioelectrical system, electroactive microorganisms oxidize organic matter and release electrons to the anode as well as hydrogen ions and CO2 (Torres et al. 2007). Those substrates are used by other microorganisms together with the electrons released by the cathode to produce hydrogen gas and subsequently methane.
In detail, three mechanisms can play a role: (1) direct bioelectrochemical methanation, (2) H2-mediated methanation also known as hydrogenotrophic methanogenesis and (3) carbon compounds-mediated methanation consisting of autotrophic acetogenesis combined with acetoclastic methanogenesis (Park et al. 2020a). The different pathways enable a high flexibility and thus, allow for maintaining a stable biogas formation process. Hence, the bioelectrical process increases the methane production rate compared to typical anaerobic systems (Zhang et al. 2018, Park et al. 2020b) and shortens stabilisation periods after shock loads (De Vrieze et al. 2018, Park et al. 2020b).
Flow scheme of the technology
The electrostimulated anaerobic reactor is an upflow anaerobic sludge blanket reactor with integrated electrodes (Fig. 1). The electroconductive anode consists of the fluidized bed made of activated carbon particles. This anode transfers the electrons via collision to a static graphite electrode with a polarized structure. The granular activated carbon is be added only once, during the start-up. However, some extra GAC injections may be necessary to compensate losses (purge, treated water). Because of their higher density than that of the biomass granules, GAC will be slightly fluidized (expected < 40%) and therefore it will be located in the lowest zone of the bioreactor.
Fig. 1 Electrostimulated anaerobic reactor (ELSAR): UASB reactor with integrated electrodes
The wastewater is pumped in the reactor at the bottom and flows to the top in a vertical cylindrical reactor. The 3-phase separator separates the gas, liquids and solids (granular activated carbon and granular sludge). The granules settle down to the bottom. The liquid stream and the biogas bubbles ensure good mixing conditions.
In the buffer tank (same height, less diameter than the reactor), the pH and the temperature of the inlet wastewater are adjusted by means of chemical addition (NaOH or HCl) and heating (Ultimate case study: 30-37°C), respectively. The biogas is collected in every separator and recirculated to add alkalinity to the inlet wastewater. On the top of the reactor, the clear water is collected.
Synergetic effects and motivation for the implementation of the technology
- Higher methane production rates compared to anaerobic digestion
The bioelectrochemical anaerobic digestion accelerates the hydrogen production due to the release of hydrogen ions from organic matter during electrolysis (Park et al. 2020b). The higher availability of hydrogen together with CO2 allows hydrogenotrophic methanogens to produce more methane due to higher process efficiencies and due to faster production rates (Park et al. 2020a).
Furthermore, An et al. 2020 showed, that electroactive bacteria rapidly enriched on the surface of an electrode as well as in the bulk solution and adapted themselves to the conditions in the reactor. Due to their contribution to methane production via the direct interspecies electron transfer pathways on the electrode and in the bulk solution, the methane production rate increased significantly (An et al. 2020).
- Shorter stabilisation periods compared to anaerobic digestion
Park et al. 2019 and Asensio et al. 2021 showed that bioelectrochemical anaerobic digestion overcomes the imbalance during shock loads or high organic loading rates between 10 kg COD/(m³*d) and 24 kg COD/(m³*d), because electroactive microorganisms oxidize organic matter and release electrons to the anode as well as hydrogen ions (H+) and CO2. In parallel, hydrogenotrophic methanogens convert rapidly the H+ using the electrons released from the cathode to H2. Hence, the pH can be regulated and an accumulation of volatile fatty acids can be decreased due to the rapid conversion of organic acids to H2 and CO2.
Technology requirements and operating conditions
As for an UASB reactor, the hydraulic retention time is decoupled from the sludge blanket residence time (Tab. 1). The reactor temperature is usually at 37 °C or 55 °C for mesophilic or thermophilic organisms, respectively. The pH of the inflow must be neutral or slightly above, because the methanogens are very sensitive to an acidic pH. The organic loading rate is usually operated between 10 and 24 kg COD/(m³*d) and the sludge content should vary between 3 and 4%. For the mixing conditions, the liquid upflow velocity plays a crucial role and is depending on the reactor geometry between 1 and 6 m/h.
For the electrodes, usually, the applied voltages range between 0.2 and 1.5 V depending on the type of substrate. The current densities also depend on the type of substrate and range between 0.45 and 9 A/(m² electrode surface area).
Tab. 1 Typical ranges for operating parameters
Parameter |
Units |
Min |
Max |
Reference |
Organic loading rate |
kg COD/(m³*d) |
10 |
24 |
|
Sludge content |
% |
3 |
4 |
|
pH |
- |
7 |
8.5 |
|
Temperature |
°C |
35 |
55 |
Deublein et al. 2008 |
Hydraulic retention time (UASB) |
h |
4 |
10 |
Deublein et al. 2008, Metcalf et al. 2013 |
Sludge blanket residence time |
d |
>30 |
||
Liquid stream velocity |
m/h |
1 |
6 |
|
Applied voltages |
V |
0.2 |
1.5 |
|
Current densities |
A/m² |
0.45 |
9.0 |
Key performance indicators
Depending on the types of substrates, the methane yields vary as shown in Tab. 2. The COD removal rates of around 86% of a bioelectrochemical anaerobic reactor are higher than those of non-electrochemical systems such as aerobic membrane bioreactors, sequencing batch reactors and anaerobic UASB reactors ranging between 70%-80% (Asensio et al. 2021).
Tab. 2 Average methane yields and COD removal efficiencies for different types of substrate in a bioelectrochemical anaerobic reactor; oDM organic dry matter
Parameter |
Unit |
Methane yield |
References |
Food waste |
m³/(kg oDM) |
0.53 |
|
Distillery wastewater |
m³/(kg oDM) |
0.48 |
|
Brewery wastewater |
m³/(kg oDM) |
0.22 |
|
|
Unit |
COD removal |
|
Brewery wastewater |
% |
85-87 |
For waste activated sludge as substrate, Cai et al. 2016 and Bo et al. 2014 observed in their laboratory experiments a two-fold higher methane yield in the bioelectrochemical system than for their conventional anaerobic digester control reactors.
Links to related topics and similar reference projects
Process/technologies |
Reference |
Enhanced biogas production due to thermal hydrolysis process (THP) |
|
Anaerobic high rate reactor (AAT) |
Case study “Shafdan” & Case study “Karmiel” (Ultimate) |
Factsheet in TEB |
Case Study applying the technology
Publications
- An, Z., Feng, Q., Zhao, R., Wang, X., Bioelectrochemical methane production from food waste in anaerobic digestion using a carbon-modified copper foam electrode, 2020
- Asensio, Y., Llorente, M., Tejedor-Sanz, S., Fernández-Labrador, P., Manchon, C., Ortiz, J.M., Ciriza, J.F., Monsalvo, V., Rogalla, F., Esteve-Núñez, A., Microbial electrochemical fluidized bed reactor (ME-FBR): An energy-efficient advanced solution for treating real brewery wastewater with different initial organic loading rates, 2021
- Bo, T., Zhu, X., Zhang, L., Tao, Y., He, X., Li, D., Yan, Z., A new upgraded biogas production process: Coupling microbial electrolysis cell and anaerobic digestion in single-chamber, barrel-shape stainless steel reactor, 2014
- Cai, W., Liu, W., Yang, C., Wang, L., Liang, B., Thangavel, S. Zechong Guo, Z., Wang A., Biocathodic methanogenic community in an integrated anaerobic digestion and microbial electrolysis system for enhancement of methane pioduction from waste sludge, 2016
- Chen, Y., Cheng, J., Creamer, K., Inhibition of anaerobic digestion process: A review, 2008
- Deublein, D., Steinhauser, A., Biogas from waste and renewable resources, 2008
- De Vrieze, J., Arends, J., Verbeeck, K., Gildemyn, S., Rabaey, K., Interfacing anaerobic digestion with bioelectrical systems: potentials and challenges, 2018
- Feng, Q., Song, Y., Yoo, K., Kuppanan, N., Subudhi, S., Lal, B., Bioelectrochemical enhancement of direct interspecies electron transfer in upflow anaerobic reactor with effluent recirculation for acidic distillery wastewater, 2017
- Liu, W., Cai, W., Guo, Z., Wang, L., Yang, C, Varrone, C., Wang, A., Microbial electrolysis contribution to anaerobic digestion of waste activated sludge, leading to accelerated methane production, 2016
- Metcalf & Eddy, Inc., Tchobanoglous, G., Stensel, H., Tsuchihashi, R. Burton, F., Wastewater Engineering: Treatment and Resource Recovery, 2013
- Naves Arnaldos, A., van den Broeke, J., Guleria, T., Bruni, C., Fantone, F., Touloupi, M., Iossifidis, D., Giménez Lorang, A., Sabbah, I., Farah, K., Baransi-Karkaby, K., Pidou, M., Reguer, A., Kleyböcker, A., Jährig, J., Vredenbregt, L., Thisgaard, P., D1.9 Start-up and intermediate results of plant operation from all case studies, 2023
- Park, J., Heo, T., Kwon, H., Shi, W., Jun, H., Effects of voltage supply on the methane production rates and pathways in an anaerobic digestion reactor using different electron donors
- Park, J., Jiang, D., Lee, B., Jun, H., Towards the practical application of bioelectrochemical anaerobic digestion (BEAD): Insights into electrode materials, reactor configurations, and process designs
- Park, J., Lee, B., Kwon, H., Park, H., Jun, H., Effects of a novel auxiliary bio-electrochemical reactor on methane production from highly concentrated food waste in an anaerobic digestion reactor, 2019
- Park, J., Lee, B., Tian, D., Jun, H., Bioelectrochemical enhancement of methane production from highly concentrated food waste in a combined anaerobic digester and microbial electrolysis cell, 2018
- Sangeetha, T., Guo, Z., Liu, W., Cui, M., Yang, C., Wang, L., Wang, A., Cathode material as an influencing factor on beer wastewater treatment and methane production in a novel integrated upflow microbial electrolysis cell (Upflow-MEC), 2016
- Torres, C., Marcus, A., Rittmann, B., Kinetics of consumption of fermentation products by anode-respiring bacteria, 2007
- Zhang, S., Chang, J., Liu, W., Pan, Y., Cui, K., Chen, X., Liang, P., Zhang, Q., Qiu, Y., Huang, X., A novel augmentation strategy to accelerate methanogenesis via adding Geobacter sulfurreducens PCA in anaerobic digestion system, 2018